Capturing Protein Folding in Real Time
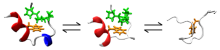
Penn Arts and Sciences Magazine: Spring/Summer 2012 issue
by Mark Wolverton
Proteins are at the very heart of life, controlling and regulating essential functions at the molecular and cellular level. One of the most important factors in how proteins operate is the way in which their molecular chains fold into different shapes. When misfolding occurs, protein function is disrupted and serious diseases can result. Yet despite our detailed knowledge of the human genome and the structure of many proteins, the folding mechanism of most proteins remains a mystery.
Feng Gai, Professor of Chemistry, explains why: "The folding occurs faster than the blink of an eye," he says. The speed and complexity of the phenomenon have, so far, made it extremely difficult to observe with the detail and resolution needed to study it. But Gai and graduate students Arnaldo Serrano and Robert Culik, along with Michelle R. Bunagan, a former graduate student in the Gai Group and now a professor at the College of New Jersey, have developed a new technique for capturing protein folding in real time—an essential first step in understanding diseases caused by protein misfolding.
Gai and his collaborators examined the Trp-cage protein using a technique called T-jump (temperature jump) infrared spectroscopy. In this technique, a protein undergoes folding triggered by a laser heating pulse, while a tunable quantum cascade laser (QCL) acts as a "camera" to observe and follow the motions of the protein's atoms. The different groups of amino acids that comprise the molecule can be compared to springs, which when excited by the QCL will vibrate at different frequencies according to their atomic mass. "Our 'camera' can detect the speed of that motion, and we can relate it to the atoms it's made of and how that segment of the protein moves," Gai says.
While the T-jump infrared spectroscopy of protein structure is nothing new in itself, the researchers have added an extra wrinkle to greatly improve the resolution of the technique. To help distinguish movements among highly similar protein bonds, the experimenters incorporated a carbon-13 isotope at a specific position within the protein. "We build upon the T-jump technique by using our tunable probe laser to look at multiple spectral signatures, some inherent to the peptide we studied, and some that we modified by incorporating a carbon-13 isotope into a specific position within the peptide," says Serrano. "By monitoring these different frequencies, we were able to observe the interesting kinetics we describe in our publication." Tuning the QCL to match the different oscillations allows separate portions of the protein components to be monitored as the peptide folds. Gai adds, "This will allow us to potentially see how a protein is misfolding in a disease."
The team plans to extend this new spectroscopy method to study other more complex proteins and their particular folding quirks. "By understanding protein folding mechanisms, we can, for example, design proteins de novo with functions they wouldn't have otherwise and understand the mechanisms of aggregation in neurodegenerative diseases," Culik notes. "To begin this process, however, we first need to do basic research to discover the rules governing how proteins fold, which we can then apply and tailor more specifically to individual diseases."